Who funds our research?
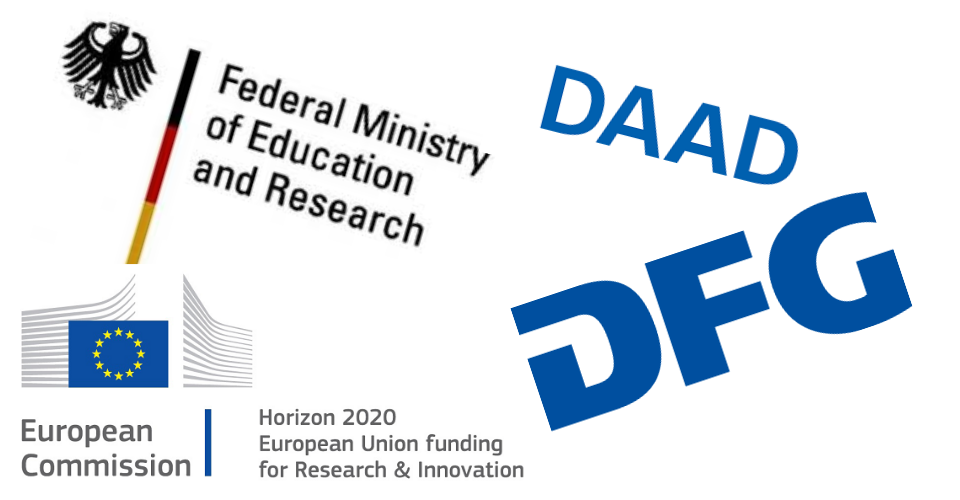
To support our research we rely on both nanitional and internation funding schemes.
On a regular basis, we are funded from the German Research Foundation (DFG), Federal Ministry of Education and Research (BMBF), Federal Minister for Economic Affairs and Energy (BMWi), German Academic Exchange Service (DAAD), and EU Framework Programmes H2020, industrial partners, and other.
The following is a list of the currently running and finished projects (continuously updating).
RUNNING
FINISHED
Running Period | Funded by | Project Number |
---|---|---|
2020.12- 2023.11 | BMWi | 03EI3029A |
Aim of this project is a positive step change in the design and the manufacturing of high performance catalysts and novel polymers for catalyst layers for application in polymer electrolyte membrane water electrolysis (PEMWE). The novel catalysts are supposed to reach higher conversion efficiencies and life times at lower cost.
One specific aim is the evluation of electroatalysts and catalyst layers. For this purpose a scanning flow cell setup coupled with inductive plasma ionization and mass spectroscopy is used. This unique setup allows to validate catalyst powder with respect to activity and stability within a short time. Another specific aim is to create optimum catalyst layers and membrane electrode assamblies based on the optimum catalyst layers by high-throughput capable manufacturing approaches (HI ERN – Thiele). Here the aim of HoKaWe is to evaluate an alternative manufacturing strategy which is based on the direct deposition of catalyst layers on porous transport layers as a substrate. This approach can be easily implemented into high-throughout manufacturing environments which is supposed to support market stimulation. All manufactured membrane electrode assemblies are characterized at HI ERN. Suitable samples are evaluated with respect of the ageing behavior in the full technical cell at IfES.
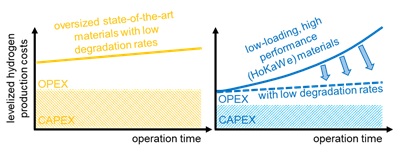
Running Period | Funded by | Project Number |
---|---|---|
2020.10- 2023.09 | BMWi | 03ETB027A |
In this project we aim at optimization of cathode catalyst layers for PEM fuel cells.
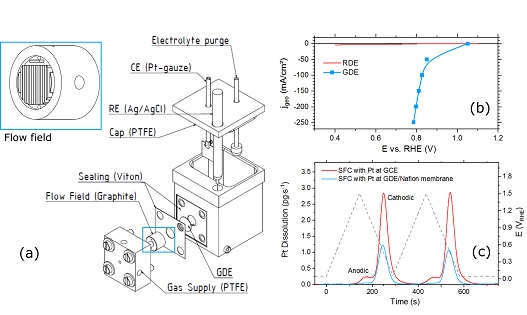
Running Period | Funded by | Project Number |
---|---|---|
2020.03-2023.02 | DFG | 413740759 |
Electrocatalysts supported on conductive high surface-area carrier materials constitute an important electrode component of electrochemical galvanic and electrolytic cell devices, such as electrolyzers or hydrogen fuel cells. The reactivity and stability of such catalyst/support couples determine the important overall performance metrics, such as cell efficiency and durability. Owing to a direct chemical interaction at their interface, catalyst and support may influence each other’s chemical reactivity, something well known in gas-solid catalysis and, there, commonly referred to as catalyst-support interaction (CSI) or more specifically, as „strong metal-support interactions (smsi)“. The concept of CSI at electrochemical solid-liquid interfaces, however, has largely remained unaddressed, yet is attracting much recent interest due to its potential to enhance both catalytic activity and electrochemical stability of electrocatalysts.
This project is designed to address fundamental scientific aspects of electrochemical CSIs at solid-liquid interfaces and explore the possibility to leverage CSIs in real electrocatalyst/support couples. Its major objective is to explore, substantiate, and quantify the chemical nature, the mechanistic origin and measurable impact of CSIs on reactivity and stability in oxide-supported Ir-based oxygen evolution (OER) electrocatalysts. Our working hypothesis is that CSIs can be tailored and leveraged to mitigate catalyst corrosion and degradation during electrolyzer operation. Using well-defined, smooth catalyst/support models, we will explore and compare a selected number of different conductive oxidic support materials and study their impact on the electronic and geometric structure as well as surface redox chemistry and catalytic OER performance of Ir-oxide catalysts with special emphasis on catalyst/support stability.
A wide variety of ex-situ and operando characterization techniques, including XAS, XPS, ICP-MS, DEMS, of well-defined surfaces and nanostructures will be employed in the course of this project. Insight in electrochemical CSIs will be transferred to nanostructured Ir based catalyst/support couples operating under catalytic OER conditions. The project team comprises three academic groups with much expertise in electrocatalysis, corrosion science and surface nanocatalysis in combination with operando spectroscopy. As a major outcome, this project will establish a deeper understanding of CSI effect in OER and its insights will aid to include CSI effect in the rational design of water splitting electrocatalysts.
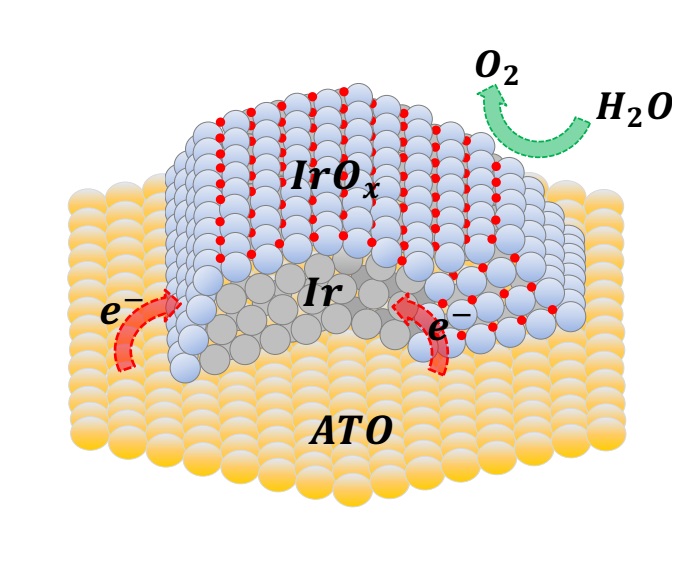
Running Period | Funded by | Project Number |
---|---|---|
2019.11-2022.10 | EU H2020 | 033RU013B |
Our vision is to enable a more distributed recycling chain, where Small-Medium Enterprises (SME), individual production plants and municipalities become the main stakeholders of the recycling process chain. This shift in the recycling paradigm hinges on the dissemination of green recycling solutions throughout the territory. The core-innovation is the development of a medium-scale, environmentally friendly and low-cost hydrometallurgical process for the extraction of noble metals from End-of-Life products (e.g. electronics waste).
Based on this technological breakthrough, we expect to trigger multiple ramifications from the technical, economic, social and environmental point of view. The cornerstone of this new hydrometallurgical recycling process is a completely new concept for noble metals chemical extraction by exploiting the so-called transient dissolution. This mechanism is a well-known degradation mechanism for all electrochemical applications and, opposite to conventional hydrometallurgy, does not require extremely aggressive conditions, but only a repeating change in surface (electro-) chemical potential.
We intend to transfer this, so far, the purely electrochemistry-related process to a chemical reactor (TRL 5) and to validate experimentally the process for real scrap, e.g., manufacturing refuse from electronics production. In order to assess the impact on the overall value chain, the project will deal with the evaluation of the recycling process in a decentralized network and the identification of the corresponding technological solutions for disassembly, pre-treatment and logistics. In conclusion, the data obtained the TRL5 reactor will serve as input data to verify the economic feasibility of our innovative chemistry and to assess the environmental benefits this new recycling scenario by means of state-of-the-art Life Cycle Costing and Analysis (LCC and LCA).
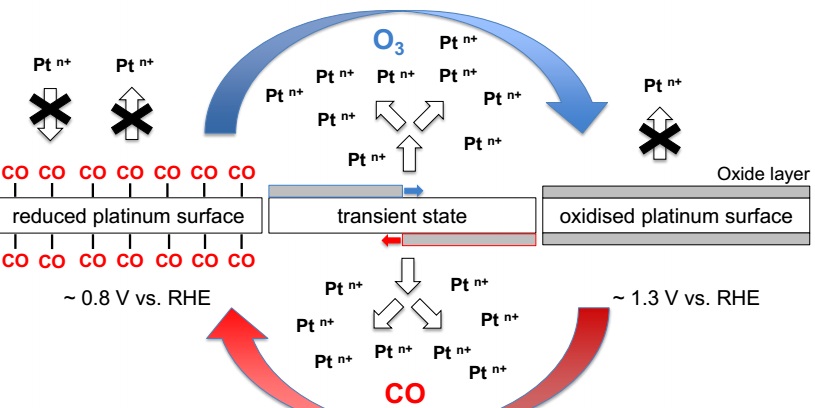
Running Period | Funded by | Project Number |
---|---|---|
2019.09-2022.08 | BMBF | P2X |
The goal of the Kopernikus project P2X is to store renewable energy in physical substances. Accordingly, the project researches possibilities for converting electricity into chemical energy. The renewable energy stored in this way can then be used in high-emissions sectors such as transport and industry, or even for heating for industrial processes, making them more environmentally friendly.
Our roal in this project is in investigation of anode catalyst layers degradation processes in proton exchange membrane water electrolysers.
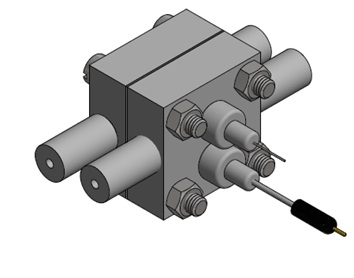
Running Period | Funded by | Project Number |
---|---|---|
2019.05-2022.06 | DFG | 418603497 |
The electrochemical oxidation of platinum and the reduction of the formed ultrathin oxide films result in Pt dissolution and surface restructuring, which is a main origin of Pt catalyst degradation in fuel cells. Although Pt oxidation has been studied for a long time, a more detailed understanding of their atomic-scale mechanisms has up to now been obtained only for the close-packed Pt(111) surface. For the accompanying Pt dissolution, microscopic models are even lacking completely.
In this project we will systematically study the oxidation, oxide reduction, and dissolution of Pt single crystal electrodes, focusing on the more open Pt(001), Pt(110), and Pt(310) surfaces. In situ surface X-ray scattering techniques will be employed for clarifying the precise atomic and nanoscale surface structure, electrochemical mass spectrometry for determining the potential- and time-dependent rates of anodic and cathodic dissolution.
We will specifically investigate (i) the mechanisms, kinetics, and reversibility of the place exchange between oxygen and Pt surface atoms in the initial stages of oxidation, (ii) the structure and structural evolution of the formed Pt oxide film, (iii) the surface restructuring caused by oxidation/reduction cycles, and (iv) how all of this influences Pt dissolution. The mass spectrometry measurements will cover a wide range of oxidation parameters, including the potential-time program, solution composition (anion species, pH, O2 content), and temperature, allowing to identify cases that are of particular fundamental interest or practical relevance for catalyst stability. With X-ray scattering we will obtain detailed structural data for a more restricted range of oxidation conditions, whose selection will be guided by the dissolution data. Together with density functional theory calculations by collaborating groups, these results should provide detailed insights on the microscopic atomic-scale mechanism. As a first test whether the developed models can be applied for understanding the stability of real catalysts, we will additionally perform complementary studies for an intermediate model system – shape-controlled Pt nanocrystals with defined facets on planar carbon supports.
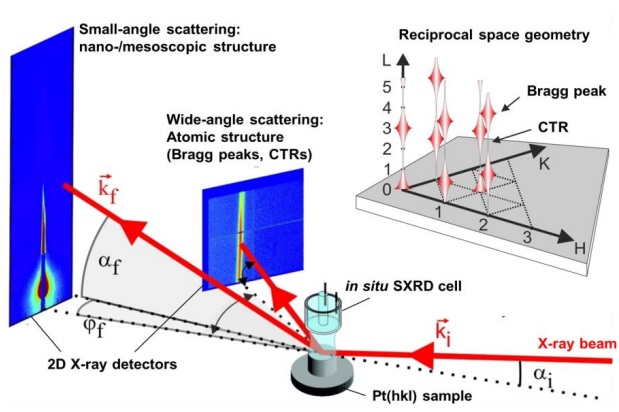
Running Period | Funded by | Project Number |
---|---|---|
2019.01- 2021.12 | DFG | 406938448 |
This project deals with the structure-performance relationships of Ir-Ru electrodes for oxygen evolution reaction during dynamic operation of proton exchange membrane water electrolysers. Whereas electrolyzers are key enablers for efficient storage of renewables within the "Energiewende", oxygen evolution is one of the most challenging and complex reactions hindering wide-spread application of this technology. The complexity grows even larger as the intermittent nature of renewable energy requires dynamic operation. For this purpose a fundamental understanding of the processes at the catalyst during dynamic oxygen evolution is required. Whereas state-of-the-art catalysts are based on pure Ir, Ir-Ru anodes show superior performance but lower stability, including changes in oxidation state, surface/bulk structure, morphology and even dissolution. The complex interaction of catalyst structure and electrochemical activity is not understood yet, but of utmost importance for enabling high performance, durable and low cost electrolyzers.
The main objective of this proposal is to gain insight into the structure-performance relationships of Ir-Ru anodes for oxygen evolution during dynamic operation. Special focus is on changes of the catalyst composition and oxidation state and their effect on reaction kinetics, and as such on catalyst activity and durability. We will elucidate, how the structure and performance changes upon full load, partial load and dynamic operation and what limits the feasible operating range. To achieve these objectives, we will develop a methodology which interlinks in situ/operando spectroscopic and spectrometric methods, which characterize catalyst state and structure, with advanced electrochemical methods and kinetic modeling techniques, which analyze and predict performance for given catalyst states.
This interdisciplinary approach includes new cells for operando X-ray spectroscopy to unravel the structure during dynamic operation and on-line inductively coupled plasma mass spectrometry and electrochemical spectroscopy setups for prescreening Ir:Ru compositions and in-depth mechanistic analysis. Results from operando spectroscopy and electrochemical characterization are combined with kinetic models that describe changes in reaction kinetics and catalyst structure and their interaction as a function of (dynamic) operating conditions. Experimentally validated kinetic models give insight into the processes at the catalyst and aid in interpreting measurements. In essence, this combination of experimental and theoretical efforts allows not only for a deeper understanding of structure-performance relationships of electrocatalysts, here exemplarily for oxygen evolution, but also for prediction of performance during dynamic operation and identification of favorable operating regimes. Moreover, the gained understanding of catalyst dynamics during oxygen evolution will pave the way for new catalysts.
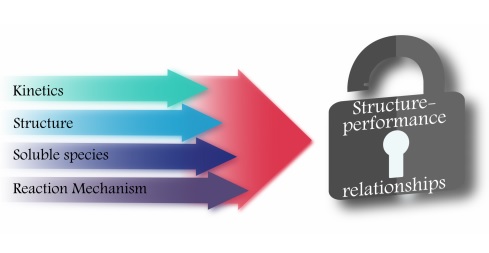
Running Period | Funded by | Project Number |
---|---|---|
2018.01-2019.12 | DAAD | 57400502 |
The overall scientific goal of this project is to gain an improved understanding of the reactive gases or liquids induced electrochemical dissolution of precious metals (PMs) with further aim of employing the collected knowledge in the design of a recycling reactor. The list of PMs that are targeted amongst others in the proposed project includes: iridium, ruthenium, rhodium, palladium, gold, and platinum as a benchmark.
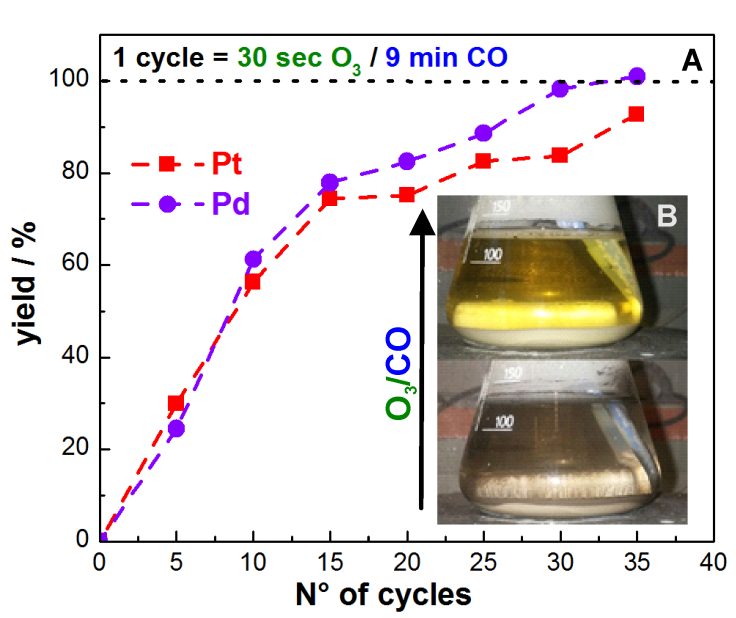
Running Period | Funded by | Project Number |
---|---|---|
2017.01 - 2020.12 | EU H2020 | 721065 |
CREATE aims at developing innovative membrane electrode assemblies for low-temperature polymer-electrolyte fuel cell (FC) and electrolyzer (EL) with much reduced cost. This will be achieved via elimination or drastic reduction of critical raw materials in their catalysts, in particular platinum group metals (PGM).
Key issues with present low-temperature FC & EL are the high contents of PGM in devices based on proton-exchange-membrane (PEM) and the need for liquid electrolytes in alkaline FC and EL. To overcome this, we will shift from PEM-based cells to 1) pure anion-conducting polymer-electrolytes and 2) to bipolar-membrane polymer electrolytes. The latter comprises anion and proton conducting ionomers and a junction. Bipolar membranes allow adapting the pH at each electrode, thereby opening the door to improved performance or PGM-free catalysts. Both strategies carry the potentiality to eliminate or drastically reduce the need for PGM while maintaining the advantages of PEM-based devices.
In strategy 1, novel anion-exchange ionomers and membranes will be developed and interfaced with catalysts based on Earth-abundant metal oxides or metal-carbon composites for the oxygen reactions, and with ultralow PGM or PGM-free catalysts for the hydrogen reactions.
In strategy 2, novel bipolar membrane designs, or designs unexplored for FC & EL, will be developed and interfaced with catalysts for the oxygen reactions (high pH side of the bipolar membrane) and with catalysts for the hydrogen reactions (low pH side). The ionomers and oxygen reaction catalysts developed in strategy 1 will be equally useful for strategy 2, while identified PGM-free and ultralow-PGM catalysts will be implemented for the hydrogen reactions on the acidic side.
Polymer-electrolyte FC & EL based on those concepts will be evaluated for targeted applications, i.e. photovoltaic electricity storage, off-grid back-up power and H2 production. The targeted market is distributed small-scale systems.
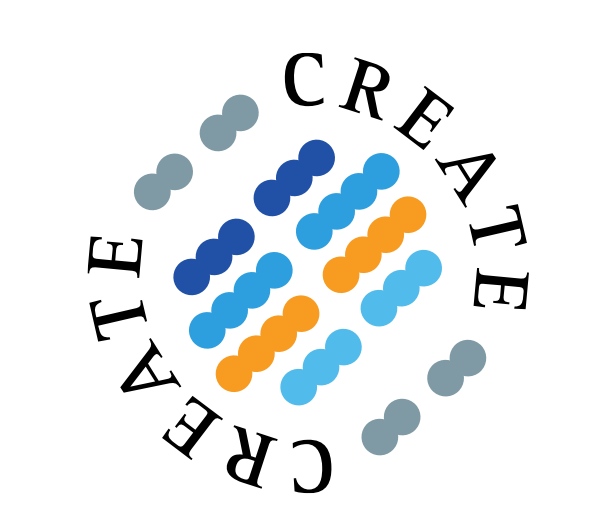