Electrochemical on-line ICP-MS
Researchers: Attila Kormanyos, Daniel Escalera, Florian Speck, Kevin Stojanovski, Konrad Ehelebe, Matej Zlatar
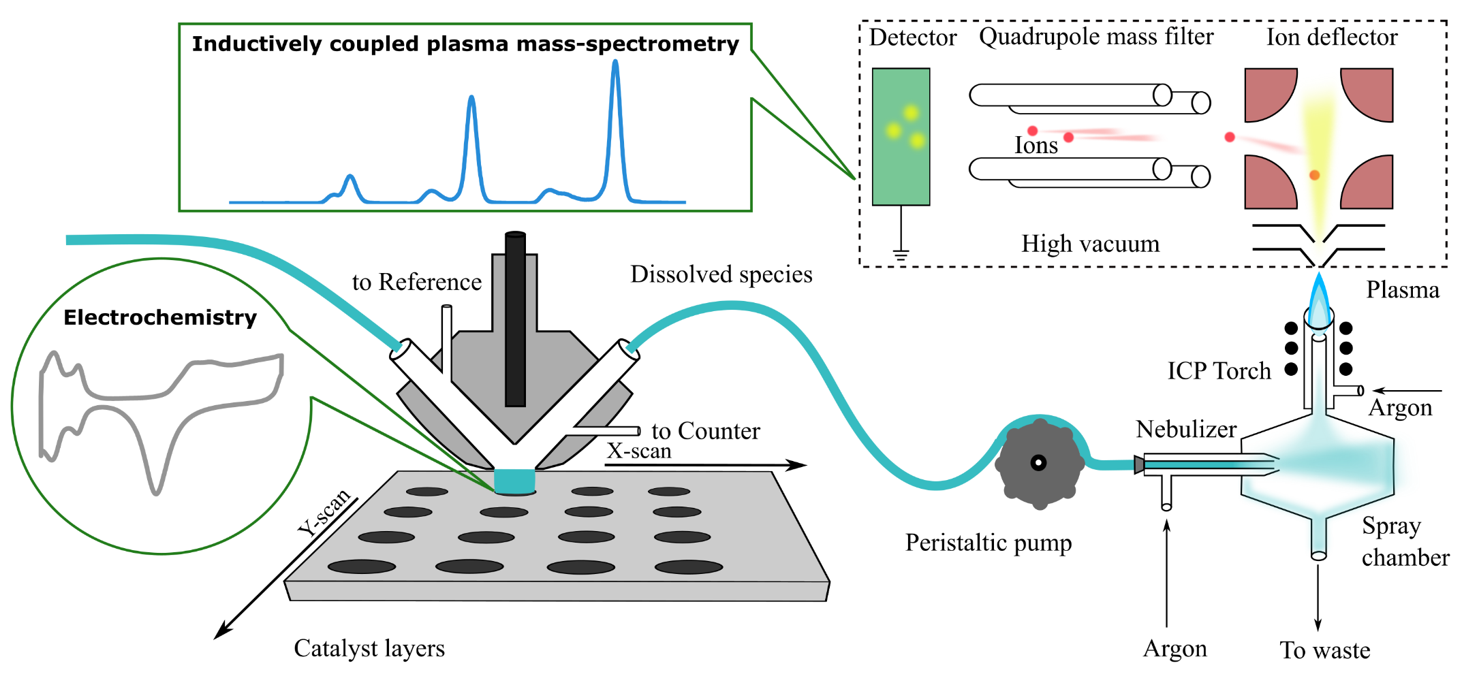
The coupling of a scanning flow cell to an inductively coupled mass spectrometer allows for the online detection of electrochemically dissolved metals. A variety of in-house cell designs allows for the application to a variety of materials. Solid metals, gradient alloys or dropcast catalyst inks are placed on a movable stage underneath the cell, allowing for rapid scanning from one measurement to the next.
While performing an electrochemical measurement, metal species are dissolved and travel downstream at a controlled flow rate via a peristaltic pump and are introduced into the ICP-MS. Sample introduction begins with the nebulizer creating a fine electrolyte mist in the spray chamber, of which a small portion enters the ICP torch plasma. The plasma is directed into an ion deflector and the ion mass composition is analyzed via a quadrupole mass filter. Detected ions create a time resolved signal of dissolution which can be directly coordinated to the applied potentiostatic or galvanostatic conditions.
Further reading:
-
Electrochemical on-line ICP-MS in electrocatalysis research
O. Kasian, S. Geiger, K.J.J. Mayrhofer, S. Cherevko*
The Chemical Record
19(10)
(2019)
2130-2142
Photo-electrochemical on-line ICP-MS
Researchers: Ken Jenewein
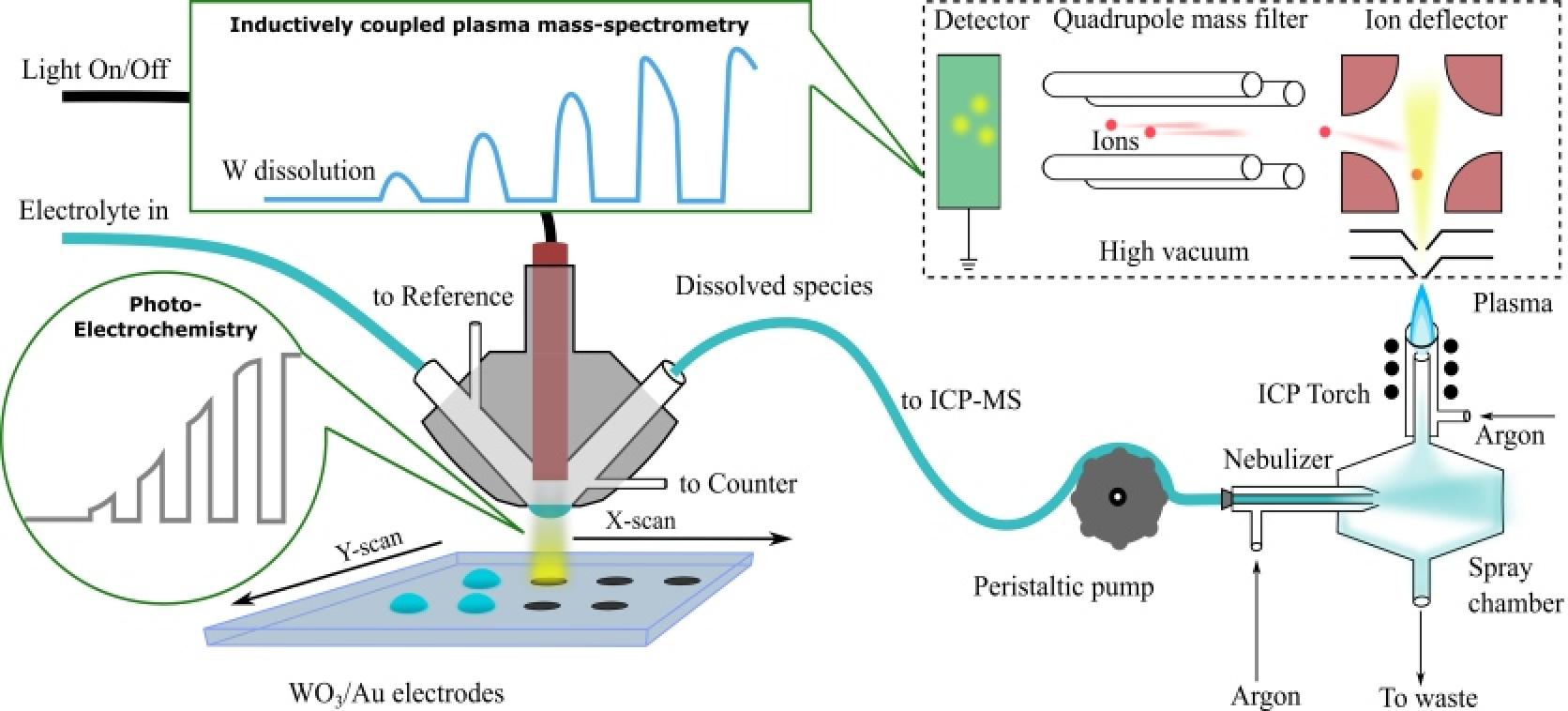
Photoelectrochemical water splitting is considered as an alternative technology to classical harvesting electricity by photovoltaics and hydrogen production water splitting in electrolyzers. Even though the technological, environmental, or economic advantage of this technology is still to be proven, the stability of photoabsorbers and electrocatalysts during photoelectrochemical water splitting is of great scientific interest. Our group recently demonstrated that even materials typically considered stable suffer from dissolution. Thus, due to the high anodic potential of the photogenerated holes and the presence of complexing sulfate ions in electrolytes, WO3 photoanodes dissolve severely during light illumination. Moreover, dissolution was found to scale mainly with anodic current.
Further investigations focus on understanding photocorrosion of photoanodes like WO3, Fe2O3, or BiVO4 and their dependence on different electrolytes, hole scavengers, and dense or molecular protective/catalytic overlayers (e.g., TiO2, Ir-molecular catalyst, or dense Ir layer)
Further reading:
-
Photocorrosion of hematite photoanodes in neutral and alkaline electrolytes
V. Benavente Llorente,* K.J. Jenewein, M. Bierling, A. Körner, A. Hutzler, A. Kormányos,* S. Cherevko*
The Journal of Physical Chemistry C
127(39)
(2023)
19687-19697
-
Electrolyte engineering stabilizes photoanodes decorated with molecular catalysts
K.J. Jenewein,* Y. Wang, T. Liu, T. McDonald, M. Zlatar, V.B. Llorente, N. Kulyk, A. Kormányos, D. Wang, S. Cherevko*
ChemSusChem
16
(2023)
e202202319
-
Dissolution of WO3 modified with IrOx overlayers during photoelectrochemical water splitting
K.J. Jenewein,* J. Knöppel, A. Hofer, A. Kormányos, B. Mayerhöfer, F.D. Speck, M. Bierling, S. Thiele, J. Bachmann, S. Cherevko*
SusMat
3(1)
(2023)
128-136
-
H. Wilson, A. Van Rooij, K.J. Jenewein, J. Knöppel, A. Kormanyos, S. Cherevko, S. Sunde, A. Erbe*
Physica Status Solidi A: Applications and Materials Science
219(11)
(2022)
2100852
-
K.J. Jenewein*, A. Kormányos, J. Knöppel, K.J.J. Mayrhofer, S. Cherevko*
ACS Measurement Science Au
1(2)
(2021)
74–81
-
Photocorrosion of WO3 photoanodes in different electrolytes
J. Knöppel,* A. Kormányos, B. Mayerhöfer, A. Hofer, M. Bierling, J. Bachmann, S. Thiele, S. Cherevko*
ACS Physical Chemistry Au
1(1)
(2021)
6–13
-
Different photostability of BiVO4 in near-pH-neutral electrolytes
S. Zhang, I. Ahmet,S.-H. Kim, O. Kasian, A.M. Mingers, P. Schnell, M. Kölbach, J. Lim, A. Fischer, K.J.J. Mayrhofer, S. Cherevko, B. Gault, R. van de Krol*, C. Scheu*
ACS Applied Energy Materials
3(10)
(2020)
9523–9527
-
S. Zhang,* M. Rohloff, O. Kasian, A.M. Mingers, K.J.J. Mayrhofer, A. Fischer, C. Scheu, S. Cherevko*
The Journal Of Physical Chemistry C
123(38)
(2019)
23410-23418
-
J. Knöppel,* S. Zhang, F.D. Speck, K.J.J. Mayrhofer, C. Scheu, S. Cherevko*
Electrochemistry Communications
96
(2018)
53-56
On-line gas MS (OLEMS/DEMS)
Researchers: Konrad Ehelebe, Moritz Geuß
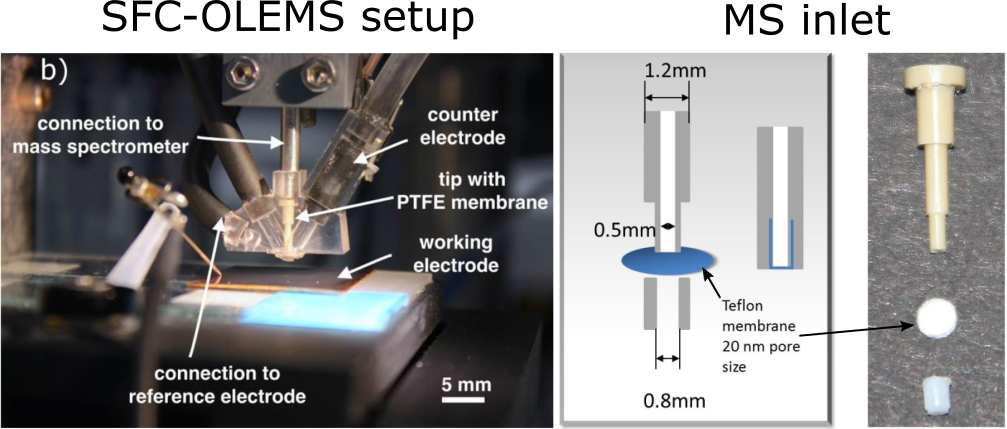
On-line electrochemical mass spectrometry (OLEMS) or differential electrochemical mass spectrometry (DEMS) is a technique used in electrochemistry for time- and potential-resolved analysis of gases or volatile species formed on the working electrode. In typical OLEMS/DEMS cells a gas transparent membrane is used to divide the electrochemical environment from the high vacuum of a mass spectrometer. Most cells are stationery. By attaching gas MS to an SFC, however, this technique can be used in the fast scanning mode. This allows utilization of SFC-OLEMS in both fundamental research (e.g. identification of IrO3 intermediate during oxygen evolution reaction on Ir electrodes) and high-throughput electrochemistry (e.g. screening CO2 reduction selectivity towards CH4 and C2H4 on CoCu gradient libraries).
Further reading:
-
The Stability-number as a metric for electrocatalyst stability benchmarking
S. Geiger,* O. Kasian, M. Ledendecker, E. Pizzutilo, A. Mingers, W.T. Fu, O. Diaz-Morales, Z. Li, T. Oellers, L. Fruchter, A. Ludwig, K.J.J Mayrhofer, M. Koper, and S. Cherevko*
Nature Catalysis
1
(2018)
508-515
-
O. Kasian,* J.-P. Grote, S. Geiger, S. Cherevko, K.J.J. Mayrhofer*
Angewandte Chemie
57(9)
(2018)
24882491
-
J-P. Grote,* A.R. Zeradjanin, S. Cherevko, K.J.J. Mayrhofer*
Review of Scientific Instruments
85
(2014)
104101-1-10401-5
-
Effect of thiol self-assembled monolayers and plasma-polymer films on dealloying
A. Pareek, G. N. Ankah, S. Cherevko, P. Ebbinghaus, K. J.J. Mayrhofer, A. Erbe, F. U. Renner*
RSC Advances
3
(2013)
6586-6595
Identical location TEM
Researchers: Daniel Escalera
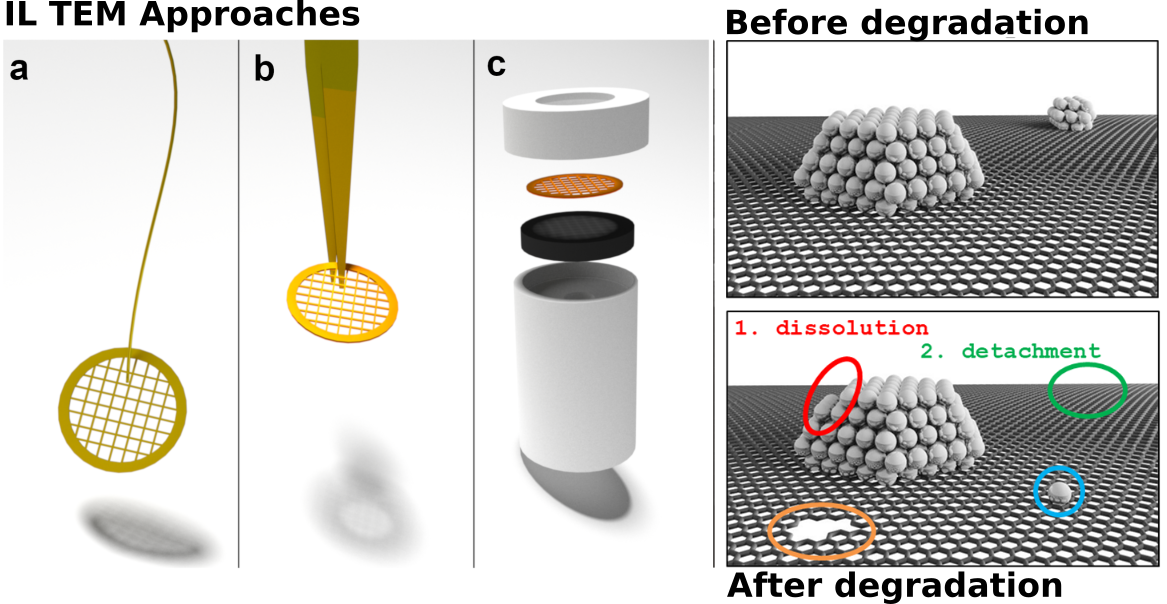
Electron microscopy is an powerful technique for observing materials on the nanoscale. As more and more electrocatalysts nanomaterials are being developed with enhanced performances, electron microscopy has become an essential technique for observing these nanocatalysts.
To perform identical location transmission electron microscopy (IL-TEM) investigations, the material of interest was first casted onto a finder TEM grid for the ease of identifying the locations upon subsequent visualizations. The sample was first visualized with the TEM at specific locations to identify its distinctive features. Specifically, the morphology, phase, elemental distribution, oxidation state distribution, and 3D distribution of the sample can be recorded.
Following the initial TEM visualization, the sample can then be loaded into an electrochemical system for performing the experiments of interest. The TEM grid can be freely suspended in the electrolyte (connected via a wire or tweezer), incorporated into an rotating disc electrode tip, or assembled into a testing fuel cell membrane electrode assembly.
After the electrochemical experiments, the TEM grid was air dried, and loaded into the TEM. The exact same location was found with the help of the finder markings, and the exact same set of techniques can be applied and the differences from that of the initial visualization documented.
Further reading:
-
Spot the difference at the nanoscale: Identical location electron microscopy in electrocatalysis
N. Hodnik* and S. Cherevko*
Current Opinion in Electrochemistry
15
(2019)
73-82
High-throughput electrochemistry
Researchers: Joanna Przybysz, Ken Jenewein
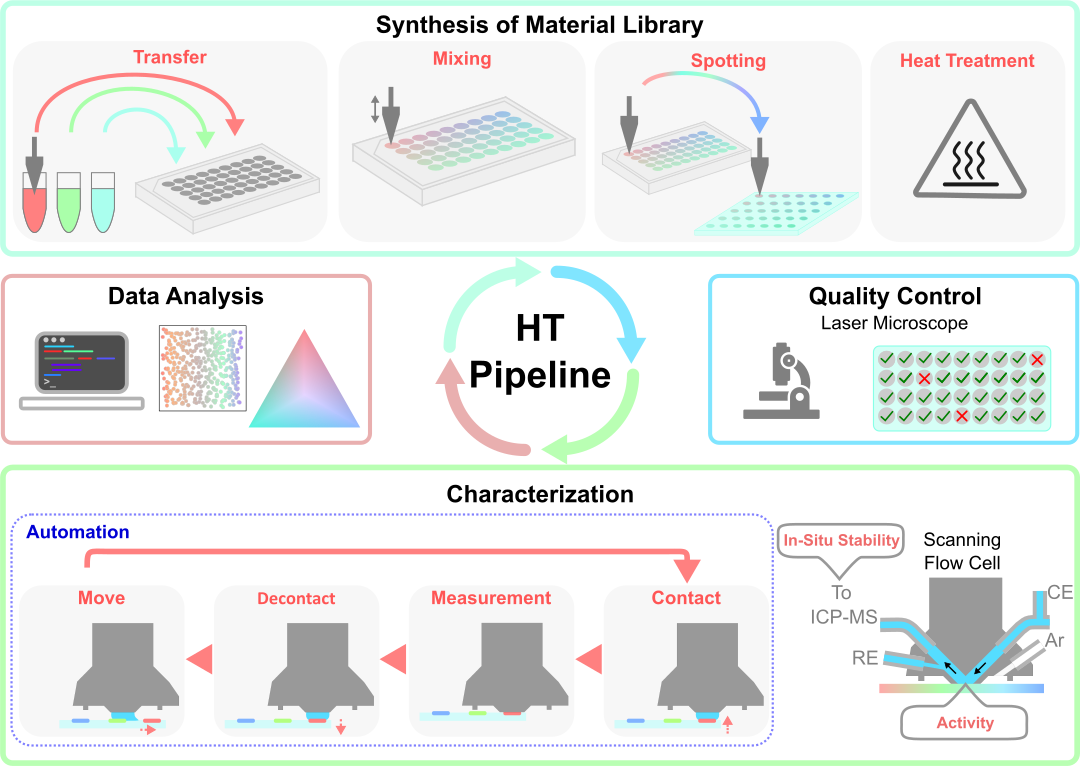
To fully make use of the versatility of our electrochemical scanning flow cell (SFC) and on-line techniques, we take various high-throughput synthesis approaches that enable automated measurements with the SFC.
-
Film synthesis utilizing an automated Nanoplotter or liquid handling robot for solution mixing and dropcasting of organic and inorganic metal complexes.
-
Thin films prepared by physical vapor deposition with the capability of introducing atomic composition gradients.
-
Electrodeposition using a modified SFC as the deposition cell.
We are working towards a fast and efficient electrochemical and physical screening method for such material libraries to preselect outstanding catalyst compositions by a mostly autonamted testing procedure. Hence, we can cut down the time spent on in-depth mechanistic analysis of catalysts, which are insignificant and hopefully even predict material properties.
The high-throughput catalyst libraries synthesis methods are combined with automated physicochemical characterization (ICP-MS, XPS, EDS, XRF, laser microscope, etc.) and fast electrochemical screening with SFC-based techniques.
With the accumulation of a larger dataset through high-throughput experiments, data science tools can become useful to aid in, for instance, the interpretation of correlating parameters or dimensionality reduction using principal component analysis (PCA) or multidimensional scaling (MDS).
Furthermore, machine learning (ML)-guided experimental planning can facilitate screening vast parameter spaces through iterative sampling. Methods such as Bayesian Optimization thus allow the finding of optimal parameter combinations (e.g., composition, processing parameters, screening parameters) without the need to check every combinatorial possibility. Aspects like the multiobjective nature of catalyst optimization (activity, stability, selectivity) or the multi-fidelity of experimental equipment can also be incorporated into Bayesian Optimization to increase the optimization performance.
Further reading:
-
K.J. Jenewein,* L. Torresi, N. Haghmoradi, A. Kormányos, P. Friederich, S. Cherevko*
Journal of Materials Chemistry A
12
(2024)
3072-3083
-
K.J. Jenewein, S. Thienhaus, A. Ludwig, A. Kormányos, S. Cherevko
Chemical Science
13
(2022)
13774-13781
-
High-throughput workflows in the service of (photo)electrocatalysis research
A. Kormanyos,* K.J. Jenewein, S. Cherevko*
Trends in Chemistry
4(6)
(2022)
475-478
-
Influence of fuels and pH on the dissolution stability of bifunctional PtRu/C alloy electrocatalysts
A. Kormanyos*, F.D. Speck, K.J.J. Mayrhofer, S. Cherevko*
ACS Catalysis
10
(2020)
10858-10870
Gas diffusion electrode half-cell
Researchers: Konrad Ehelebe, Moritz Geuß, Yu-Ping Ku
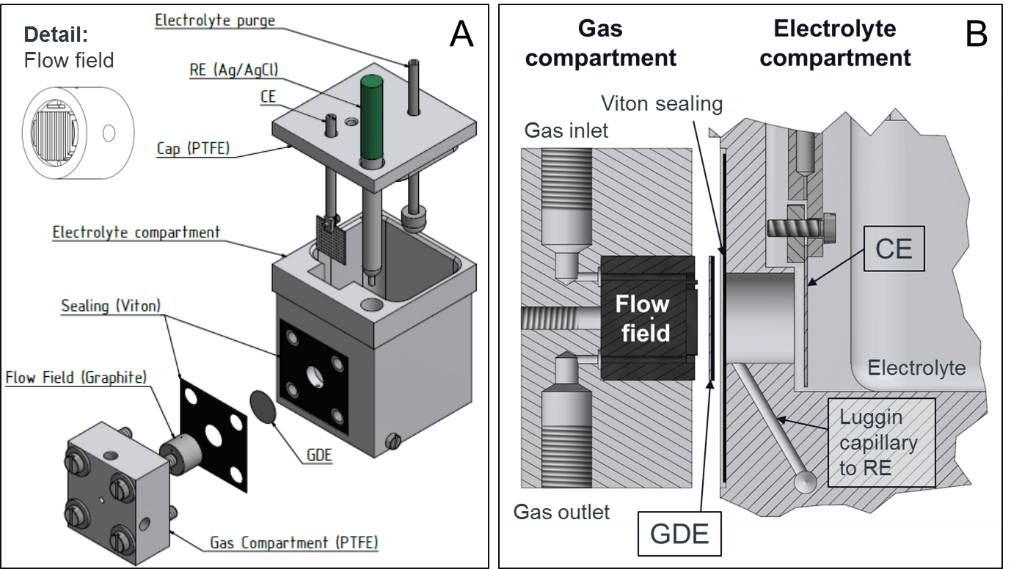
In electrochemical three-phase reactions, such as the fuel cell reactions or CO2-reduction, research in aqueous electrolytes (RDE, SFC i.e.) is limited due to diffusion limitation of dissolved educts to the electrode surface. To evaluate electrocatalysts for those reactions in high-current ranges, which are close to realistic application conditions, different techniques have to be considered.
With a gas diffusion electrode (GDE) set-up it is possible to combine the advantages of fundamental methods such as RDE (only small amount of catalyst needed for testing, simple cell configuration enables fast, low-cost and well-comparable testing) with those of more applied catalyst testing methods like MEA-testing (large potential window enabled by high mass transport, realistic catalyst layers and loadings). Thereby the activity of electrocatalysts in a current density range up to 2 A cm-2 can be evaluated.
Efforts are made to combine GDE also with SFC to track catalyst dissolution also in high-current ranges.
Further reading:
-
K. Ehelebe*, T. Ashraf, S. Hager, D. Seeberger, S. Thiele, S. Cherevko*
Electrochemistry Communications
116
(2020)
106761
-
K. Ehelebe,* D. Seeberger, M.T.Y. Paul, S. Thiele, K.J.J. Mayrhofer, S. Cherevko*
Journal of The Electrochemical Society
166(16)
(2019)
F1259-F1268
Electrochemical methods
Researchers: Attila Kormanyos, Daniel Escalera, Florian Speck, Julius Knöppel, Ken Jenewein, Kevin Stojanovski, Konrad Ehelebe, Matej Zlatar, Moritz Geuß, Yu-Ping Ku
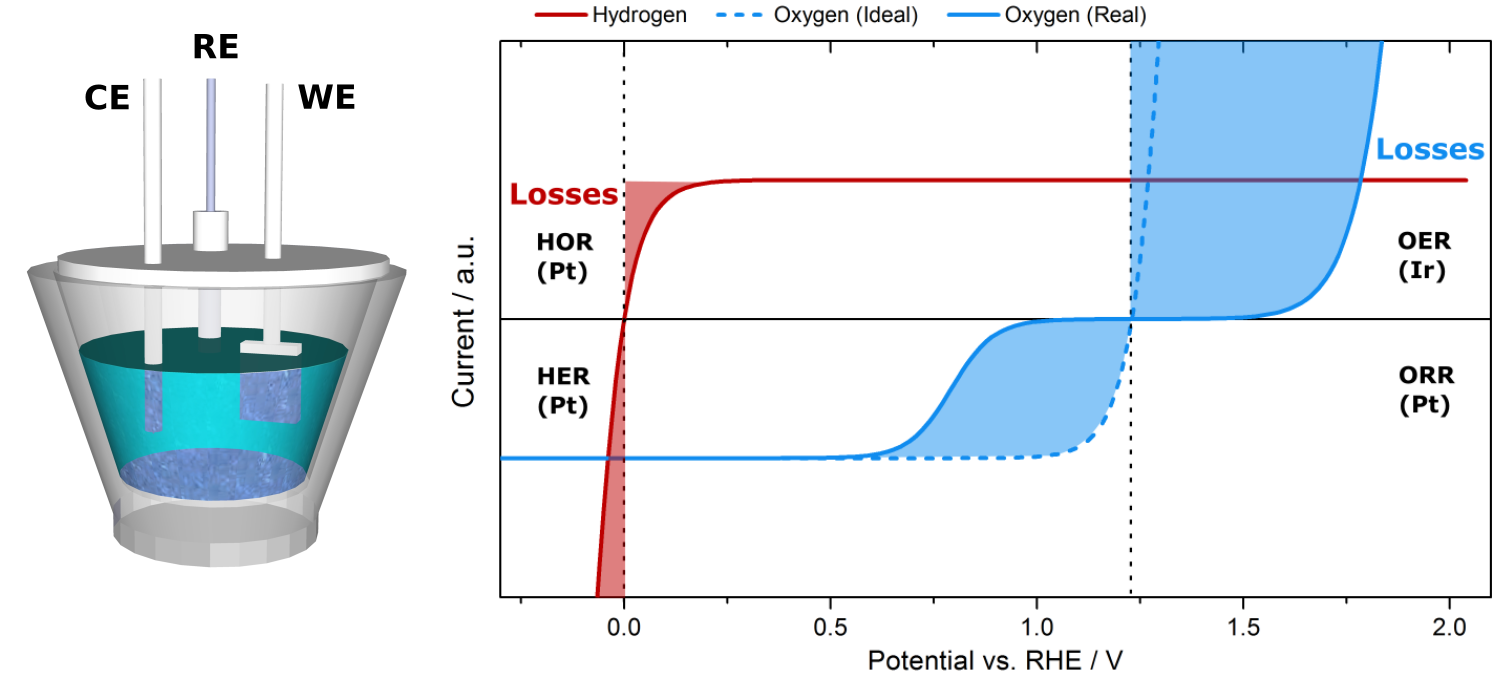
Due to their simplicity and robustness, in some cases electrochemical methods can be advantageous to the coupled techniques. In our research we distinguish several cells in which such methods can be applied:
- Classical glass or Teflon three electrode cells in fundamental research
- Scanning flow cells (SFC) for fast screening of catalyst libraries
- Cells for long-term electrochemical measurements, e.g. H-cell with separated cathode and anode compartments
- Hybrid aqueous electrolyte cells with gas diffusion electrodes.
The most used methods are cyclic voltammetry (CV), linear sweep voltammetry (LSV), chronoamperometry (CA), and electrochemical impedance spectroscopy (EIS). Using a homemade LabVIEW software different combinations of these methods can be created. This is especially useful in the long-term fully automated measurements.