Low temperature fuel cells
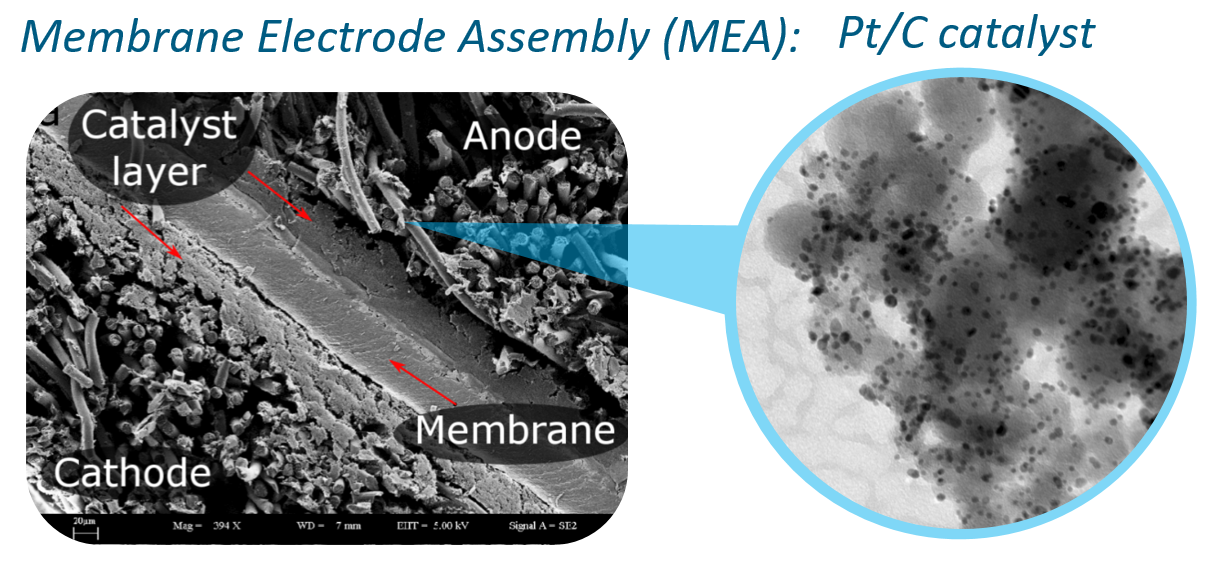
We investigate catalysts for acidic proton exchange membrane (PEM) and alkaline anion exchange membrane (AEM) fuel cell (FC) research from the fundamental to the applied level, mainly covering the low-temperature range. The stability and activity of Pt- and Pt-based oxygen reduction reaction (ORR) catalysts constitute one of the group's core research directions. Besides that, we investigate the effect of the catalyst layer composition on stability and activity to understand the interaction between the catalyst, the support, and the ink. In this, we rely on a set of methods and techniques available in the group, including high-throughput catalyst screening for fast discovery of the more advanced catalysts, online ICP-MS for time- and potential-resolved analysis of dissolution kinetics, identical location TEM to track degradation on the nanoscale, etc. and continuously are developing new methods to move into the direction of high-temperature applications, for fundamental and applied studies.
- Mechanism of platinum oxidation and dissolution – research at platinum single crystals;
- Effect of Pt particle size and Pt density in the catalyst layer on the stability – research at 2D and 3D model catalyst systems;
- The effect of ink composition on the catalyst stability and activity: ionomer, support and membrane
- Developing techniques for high-temperature, high-current applications to optimize throughput
- Activity and stability studies for Pt-based alloys for the ORR in acidic media, including PtAu, PtNiAu and other bimetallic alloys with non-noble metals.
- Modification of Pt model surfaces and alloys with organic molecules and ionic liquids for improving the activity and/or stability towards the ORR
Also, non-PGM (platinum group metals) catalysts are within our group's research interests. The most promising non-PGM ORR catalyst is Fe-N-C. In collaboration with specialists in Fe-N-C synthesis and characterization, we investigate the degradation of Fe-N-C in both PEM and AEM environments.
Further reading:
-
P. Lauf*, V. Lloret, M. Geuß, C. Collados, M. Thommes, K. Mayrhofer, K. Ehelebe, S. Cherevko*
Journal of The Electrochemical Society
170
(2023)
064509
-
Driving force of the initial step in electrochemical Pt(111) oxidation
T. Fuchs, V. Briega-Martos, J.O. Fehrs, C. Qiu, M. Mirolo, C. Yuan, S. Cherevko, J. Drnec, O.M. Magnussen, and D.A. Harrington*
The Journal of Physical Chemistry Letters
14
(2023)
3589-3593
-
Optimal Pt–Au alloying for efficient and stable oxygen reduction reaction catalyst
X. Xie, V. Briega-Martos, R. Farris, M. Dopita, M. Vorokhta, T. Skála, I. Matolínová, K. Neyman, S. Cherevko, I. Khalakhan*
ACS Applied Materials & Interfaces
15(1)
(2023)
1192–1200
-
Oxygen reduction reaction in alkaline media causes iron leaching from Fe-N-C Electrocatalysts
Y.-P. Ku*, K. Ehelebe, A. Hutzler, M. Bierling, T Böhm, A. Zitolo, M. Vorokhta, N. Bibent, F. Speck, D. Seeberger, I. Khalakhan, K. Mayrhofer, S. Thiele, F. Jaouen*, S. Cherevko*
Journal of the American Chemical Society
144(22)
(2022)
9753–9763
-
K. Ehelebe,* N. Schmitt, G.Sievers, A.W. Jensen, A. Hrnjić, P.C. Jimenez, P. Kaiser, M. Geuß, Y.-P. Ku, P. Jovanovič, K.J.J. Mayrhofer, B. Etzold, N. Hodnik, M. Escudero-Escribano, M. Arenz, S. Cherevko*
ACS Energy Letters
7
(2022)
816-826
-
K. Ehelebe, D. Escalera-López, S. Cherevko
Current Opinion in Electrochemistry
29
(2021)
100832
-
Platinum dissolution in realistic fuel cell catalyst layers
K. Ehelebe∗, J. Knöppel, M. Bierling, B. Mayerhöfer, T. Böhm, N. Kulyk, S. Thiele, K.J.J. Mayrhofer, S. Cherevko∗
Angewandte Chemie International Edition
60(16)
(2021)
8882-8888
Low-temperature electrolyzers
Researchers: Carolin Igel, Maja Milosevic, Matej Zlatar, Moritz Geuß
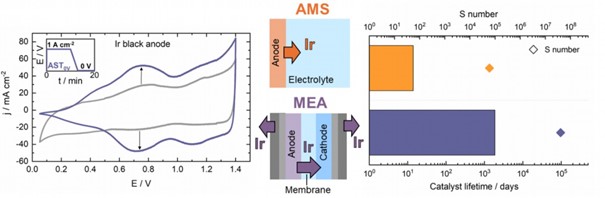
In our electrochemical hydrogen production (water electrolysis) activities, we concentrate on both acidic proton exchange membrane water electrolysis (PEMWE) and alkaline anion exchange membrane water electrolysis (AEMWE) research.
PEMWE plays an important role in the developing sustainable energy infrastructure based on the renewable energy sources and green hydrogen. However, its implementation on a TW scale is hindered by the usage of Ir as the anode catalyst for the sluggish oxygen evolution reaction (OER), since Ir is one of the most scarce metals with the annual product of ~ 7 t/year. Deep understanding on the Ir degradation processes taking place within an electrolyzer cell are of paramount importance for the development of the mitigation strategies focused on lowering the currently used state-of-the-art Ir loadings of 1-2 mg cm-2 by an order of magnitude. Here we study Ir dissolution and its subsequent transport through the system under intermittent operation. By varying the stressors within the applied electrochemical protocols, different dissolution pathways of the anode catalyst layer (ACL) are observed. Furthermore, we perform comparative studies on the Ir stability obtained in aqueous and solid electrolyte systems with the goal to determine the discrepancy between the two and bridge the gap between fundamental and applied studies in the field of PEMWE.
The new emerging technology that combines the advantageous design of a PEM electrolyzer and the usage of Earth-abundant materials as catalysts is the AEMWE. Application of Fe and Ni-based anode catalysts for OER in alkaline media presents a promising replacement of Ir and here we study the stability and durability of these materials. Further efforts are also being placed on the development of a gas diffusion electrode (GDE) setup that would allow for faster evaluation of the OER catalyst in an aqueous electrolyte setup while employing catalyst layers used in a single cell electrolyzer.
Further reading:
-
M. Geuß,* M. Milosevic, M. Bierling, L. Löttert, D. Abbas, D. Escalera-López, V. Lloret, K. Ehelebe, K.J.J. Mayrhofer, S. Thiele, S. Cherevko*
Journal of The Electrochemical Society
170
(2023)
114510
-
M. Milosevic*, T. Böhm, A. Körner, M. Bierling, L. Winkelmann, K. Ehelebe, A. Hutzler, M. Suermann, S. Thiele, and S. Cherevko*
ACS Energy Letters
8
(2023)
2682-2688
-
J. Knöppel, M. Möckl, D. Escalera-López, K. Stojanovski, M. Bierling, T. Böhm, S. Thiele, M. Rzepka, S. Cherevko
Nature Communications
12
(2021)
2231
-
The Stability-number as a metric for electrocatalyst stability benchmarking
S. Geiger,* O. Kasian, M. Ledendecker, E. Pizzutilo, A. Mingers, W.T. Fu, O. Diaz-Morales, Z. Li, T. Oellers, L. Fruchter, A. Ludwig, K.J.J Mayrhofer, M. Koper, and S. Cherevko*
Nature Catalysis
1
(2018)
508-515
-
Atomic-scale insights into surface species of electrocatalysts in three dimensions
T. Li, O. Kasian, S. Cherevko, S. Zhang, S. Geiger, C. Scheu, P. Felfer, D. Raabe, B. Gault,* K.J.J. Mayrhofer*
Nature Catalysis
1(4)
(2018)
300-305
-
O. Kasian,* J.-P. Grote, S. Geiger, S. Cherevko, K.J.J. Mayrhofer*
Angewandte Chemie
57(9)
(2018)
24882491
High-throughput electrochemistry
Researchers: Ken Jenewein
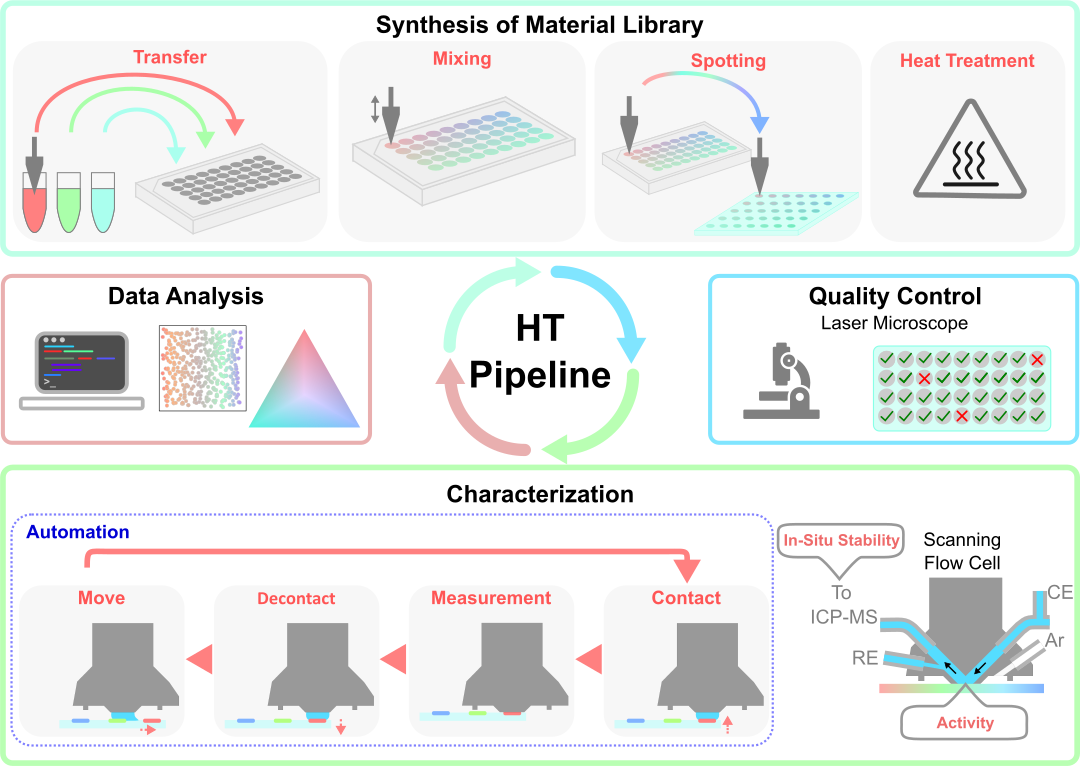
The vast majority of the electrocatalysts currently used in technologically important processes are still far away from been optimal. As most of them are composed of expensive and rare noble metals, there is a high economical reasoning for replacing current catalysts with more abundant materials. Moreover, many reactions still lack a catalyst which would provide desired performance in term of activity, selectivity, and stability. Thus, design and synthesis of novel electrocatalysts and optimization of state-of-the-art electrocatalysts occupies a significant part of the modern electrocatalysis research.
Since theoretical tools for prediction of optimal catalysts are still to be developed, we still rely on the trial-and-error approach. Although, knowledge accumulated in fundamental studies is used as a guidance. In this situation, combinatorial approaches of catalysts synthesis and characterization become an important tool in discovery of novel electrocatalysts.
Currently, our group pursue three directions in synthesis of high-throughput catalyst libraries:
- Physical vapour deposition (PVD) of gradient libraries: model flat surfaces are prepared using two and more sources (sputtering targets, e-beam evaporated materials). Representative examples are Ir-Ru libraries for OER in PEMEL;
- Electrodeposition of material libraries: model flat to applied porous surfaces can be obtained by controlling electrodeposition parameters. Combinatorial mode is realized by using scanning flow cell (SFC) with two and more electrolyte sources and electrolyte premixing. Representative example is NiFe:Au and NiFe:Ru libraries for OER in AEMEL;
- Ink-jet printing of metal precursors with additional thermal treatment: applied particulate surfaces of high-surface-area are prepared by parallel and/or consecutive two and more metal salt precursors printing. Representative examples are Ni-based alloys for HOR in AEMEL.
The high-throughput catalyst libraries synthesis methods are combined with automated physicochemical characterization (XPS, EDS, etc) and fast electrochemical screening with SFC based techniques.
Further reading:
-
O. Kasian,* S. Geiger, M. Schalenbach, A. Mingers, A. Savan, A. Ludwig, S. Cherevko, K.J.J. Mayrhofer*
Electrocatalysis
9
(2018)
139-145
-
On the origin of the improved ruthenium stability in RuO2-IrO2 mixed oxides
O.Kasian,* S. Geiger, P. Stock, G. Polymeros, B. Breitbach, A. Savan, A. Ludwig, S. Cherevko, and K.J.J. Mayrhofer*
Journal of The Electrochemical Society
163(11)
(2016)
F3099-F3104
-
G.P. Keeley,* S. Cherevko, K.J.J. Mayrhofer*
ChemElectroChem
3(1)
(2016)
51-54
Photoelectrochemistry
Researchers: Attila Kormanyos, Julius Knöppel, Ken Jenewein
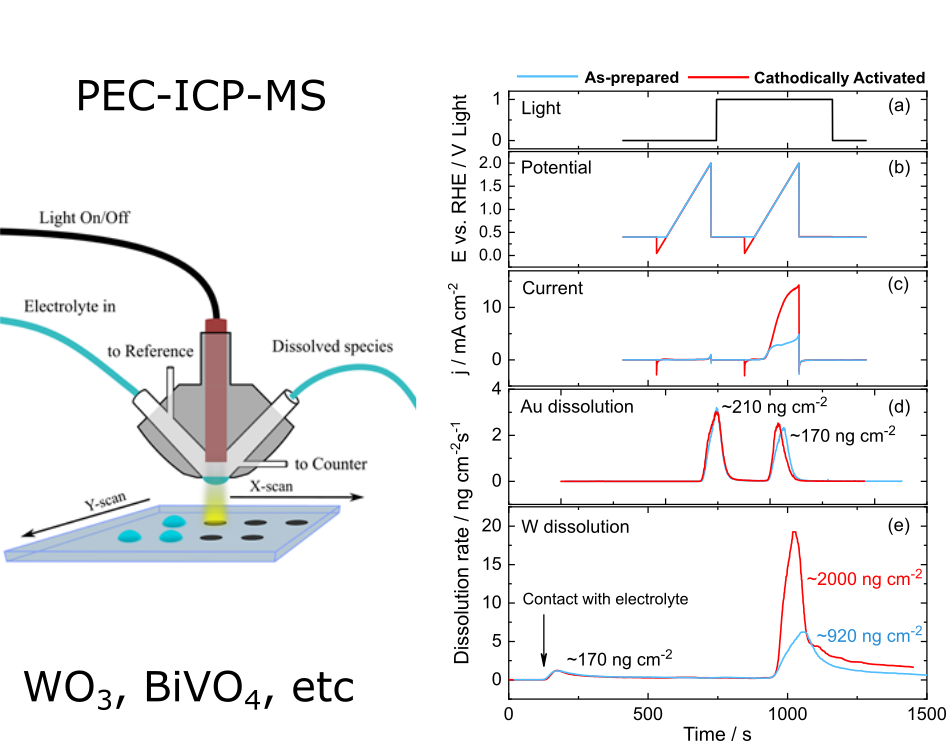
Photoelectrochemical water splitting is considered as an alternative technology to classical harvesting electricity by photovoltaics and hydrogen production water splitting in electrolyzers. Even though technological, environmental or economical advantage of this technology is still to be proven, stability of photoabsorbers and electrocatalysts during photoelectrochemical water splitting is of great scientific interest. Our group recently demonstrated that even materials typically considered as stable, suffer from dissolution. Thus, due to high anodic potential of the photogenerated holes and presence of complexing sulphate ions in electrolyte, WO3 photoanodes dissolve severely during light illumination. Moreover, dissolution was found to scale with main anodic current. Currently, we concentrate at understanding WO3 photocorrosion and its dependence on different electrolytes, presence of hole scavengers in electrolyte, presence of electrocatalyst such as Ir.
Another promising photoanode is BiVO4. In collaboration with MPIE (Germany) we investigate degradation of BiVO4 in different electrolytes.
Further reading:
-
Different photostability of BiVO4 in near-pH-neutral electrolytes
S. Zhang, I. Ahmet,S.-H. Kim, O. Kasian, A.M. Mingers, P. Schnell, M. Kölbach, J. Lim, A. Fischer, K.J.J. Mayrhofer, S. Cherevko, B. Gault, R. van de Krol*, C. Scheu*
ACS Applied Energy Materials
3(10)
(2020)
9523–9527
-
S. Zhang,* M. Rohloff, O. Kasian, A.M. Mingers, K.J.J. Mayrhofer, A. Fischer, C. Scheu, S. Cherevko*
The Journal Of Physical Chemistry C
123(38)
(2019)
23410-23418
-
J. Knöppel,* S. Zhang, F.D. Speck, K.J.J. Mayrhofer, C. Scheu, S. Cherevko*
Electrochemistry Communications
96
(2018)
53-56
Noble metals recycling
Researchers: Kevin Stojanovski, Valentín Briega Martos
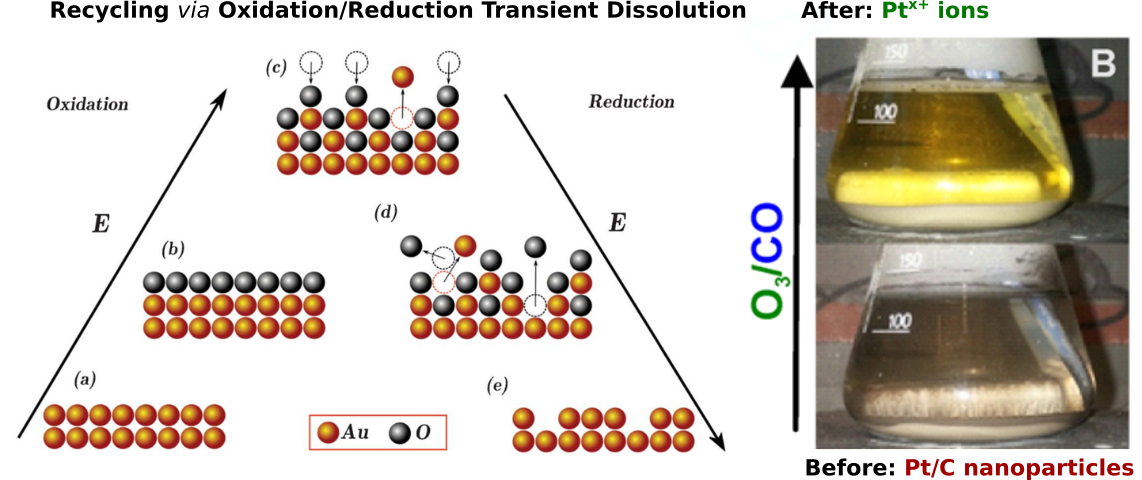
Disruptive in most electrochemical processes, dissolution can also be useful. The so-called transient dissolution which takes place during noble metals oxidation and reduction can be employed in recycling of noble metals. In this process, metal oxidation and reduction is achieved by repetitive enrichment of an electrolyte with oxidative, e.g. ozone, and reductive, e.g. hydrogen, gases or liquids. Unlike traditional hydrometallurgical processes, recycling via transient dissolution can be performed at relatively mild conditions, i.e. low base or acid concentration, room temperate, etc.
Our group concentrates on the fundamental understanding of processes taking place at electrolyte/electrode interface during dissolution. In this case our goal is to maximize the dissolution yield and selectivity. We aim at developing optimal green solutions in recycling of the platinum group metals (PGM) and noble metals like Au and Ag. A DAAD funded program is devoted to this research.
Further reading:
-
N. Hodnik,* C. Baldizzone, G. Polymeros, S. Geiger, J-P. Grote, S. Cherevko, A. Mingers, A. Zeradjanin, K.J.J. Mayrhofer*
Nature Communications
7
(2016)
13164
Fundamental research on electrochemical stability of materials
Researchers: Florian Speck
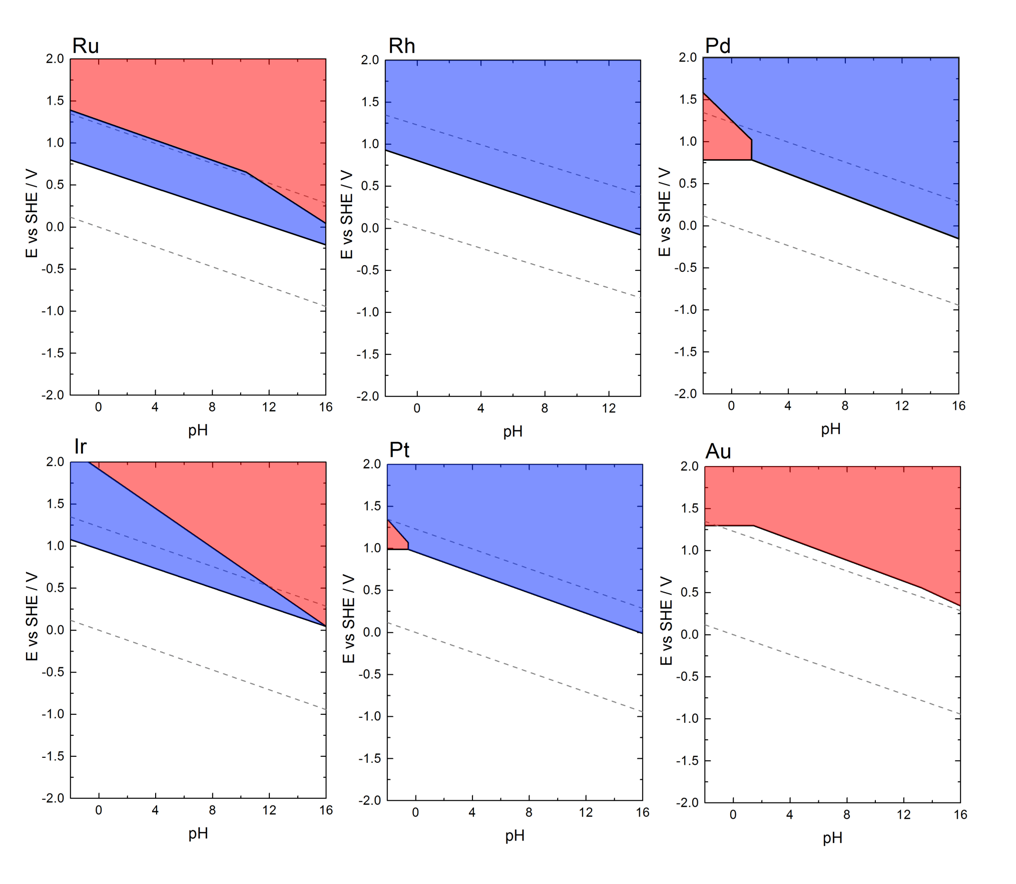
Exposed to an electrochemical environment and in response to this environment, most of technologically important materials tend to degrade. Classical example is the corrosion of unprotected steel in air. Corrosion takes also place in the electrochemical energy conversion technologies. Electrocatalyst dissolution, viz. Pt in proton exchange membrane (PEM) fuel cell (FC) and Ir in water electrolysis (EL), is a well-known degradation process. In order to understand mechanisms governing catalysts dissolution we focus on the fundamental research on model systems (single- and poly-crystalline electrodes).
Unlike research topics described more in detail in other topical sections, here we concentrate on understanding oxidation and dissolution in general. Our new results on dissolution kinetics complement the existing thermodynamic data. In our research, we aim at discovering stability descriptors and their further application in designing more stable materials, independent on technology. Our preliminary on-line ICP-MS results reveal that materials tendency towards oxidation/passivation and dissolution can be predicted based on the strength of metal-metal and/or metal-oxygen bonds.
Further reading:
-
Dissolution of platinum single crystals in acidic medium. Invited. Special Issue: Electrocatalysis.
D.J.S. Sandbeck,* O. Brummel, K.J.J. Mayrhofer, J. Libuda, I. Katsounaros, S. Cherevko*
ChemPhysChem
20(22)
(2019)
2997-3003
-
The electrochemical dissolution of noble metals in alkaline media
M. Schalenbach,* O. Kasian, M. Ledendecker, F.D. Speck, A. Mingers, K.J.J. Mayrhofer, S. Cherevko*
Electrocatalysis
9
(2018)
153-161
-
A comparative study on gold and platinum dissolution in acidic and alkaline media
S. Cherevko*, A.R. Zeradjanin, G.P. Keeley, K.J.J. Mayrhofer*
Journal of The Electrochemical Society
161(12)
(2014)
H822-H830
-
Dissolution of noble metals during oxygen evolution in acidic media
S. Cherevko*, A.R. Zeradjanin, A.A. Topalov, N. Kulyk, I. Katsounaros, K.J.J. Mayrhofer*
ChemCatChem
6
(2014)
2219-2223